The impact of sandstone discontinuities on underground H2 storage capacity
Doug Smith
Supervisors: Edward Hough, Andreas Busch, Kamaljit Singh, Daniel Arnold and Oliver Wakefield
My current research examines how small features (mm to metre-scale) within sandstone rock potentially affect large-scale (km-scale) underground hydrogen storage (UHS).
Hydrogen has the potential to help with the energy transition, balancing renewable energy supply with seasonal demand. With applications such as industrial processes (including production of ammonia or steel), transportation, reconversion to electricity and domestic heating in some circumstances, hydrogen provides a method of storing, transporting and utilising the low/zero-carbon energy we can produce (IEA 2023). As a result, many governments have adopted hydrogen as part of their future energy mix (de Coninck et al. 2018; AusGov 2019; UKGov 2021; IEA 2021; USGov 2021).
To achieve a global impact hydrogen needs to be stored at large-scale (TWh capacity), requiring large, km-scale reservoirs. Sandstone reservoirs have been utilised for gas storage for over a century and have even stored hydrogen (as a constituent of town gas). This resource is currently being explored as an option for large-scale hydrogen storage and one demonstration project in Austria has started to inject 100% hydrogen into a depleted gas field (RAG 2023).
Efficient UHS
For hydrogen storage to be cost-effective, increasing the likelihood and scope of its implementation, as much of the injected hydrogen needs to be recovered as possible. The energy required to store and recover the hydrogen and the purity of the produced hydrogen also impacts upon cost-effectiveness. My project studies how some of the features (deformation bands), often found within the most porous, and therefore potentially most suitable, sandstone affects the injection and recovery of hydrogen (Wakefield et al., 2022; Smith et al, 2024).
Sandstone and the provenance of deformation bands
I am studying the Triassic Sherwood sandstone, which is prevalent across large parts of the UK, the East Irish Sea Basin and the North Sea and is a significant repository for oil and gas (Griffiths et al., 2016; Medici et al., 2018). Within rock there are zones which have been subjected to stress and strain. If the forces are sufficient, faults and fractures will occur. However, forces that are not sufficient to break the rock may still deform it by moving or crushing the grains, altering the permeability of the rock in those zones. Deformation bands are more prevalent within aeolian sandstone, which intrinsically is well-sorted, relatively porous and considered to be good host rock for reservoirs (Wakefield et al 2022). Zones of deformation bands may extend up to hundreds of metres, with permeabilities several orders of magnitude lower than the main reservoir rock (Medici et al., 2019; Pourmalek et al. 2021). My supervisors and I visited a number of outcrops around the Wirral peninsular, where I observed deformation bands in the wild! Zones of deformation are below seismic detection, one of the main surveying techniques for underground reservoirs, making it important to understand their impact upon H2 flow in the subsurface.
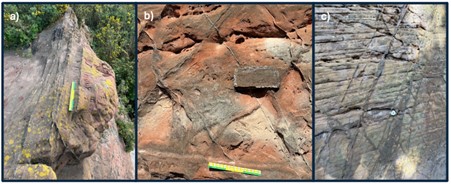
Figure 1 Three examples of deformation bands in the Sherwood sandstone from a) Thurstaston Common, b) Grange Hill and c) Bidston Court Gardens on the Wirral, with ruler and hand lens for scale.
Project aim
To model the effects of the deformation bands at metre to kilometre-scale to understand their impact upon UHS. There are 2 components to this:
- Experimental – mm-scale fluid flow experiments where hydrogen and brine are injected into a sample containing a deformation band. CT images are obtained to investigate the flow pathways and if there are any blockages or fluid traps.
- Modelling – the outputs from the experiment will be synthesised with results from other studies and scaled up to create a large-scale model that can be interrogated.
Samples
I obtained aeolian sandstone samples (Frodsham member of the Sherwood Sandstone Group) containing deformation bands from the Saughall Massie borehole (depth – 150 m), also in the Wirral (see map below).
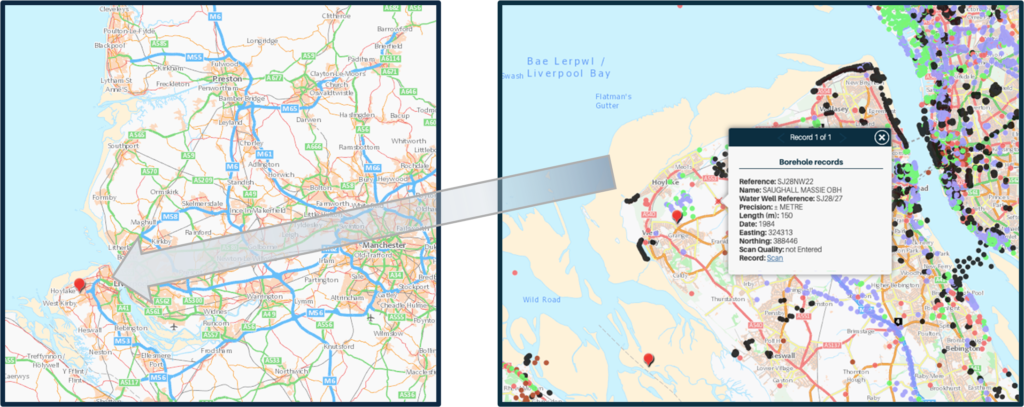
Figure 2 Location of the Saughall Massie borehole.
A number of samples have been taken from the borehole for permeability measurements, scanning electron microscope (SEM) analysis and the fluid flow experiment.
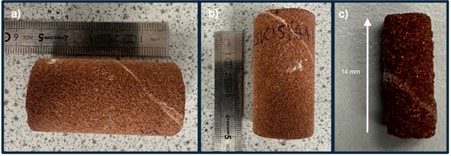
Figure 3 a) and b) different aspects of one of the 1” diameter core plug samples from the Frodsham Member of the Helsby Sandstone Formation (from the Saughall Massie borehole) containing a deformation band. c) the 6mm diameter mini-core plug, drilled vertically through the deformation band in the core plug sample shown, that was used for the fluid flow experiments.
As part of the sampling, a thin section was acquired that bisected the deformation band. It was used to understand the structure and components of the deformation band and the surrounding intact rock.
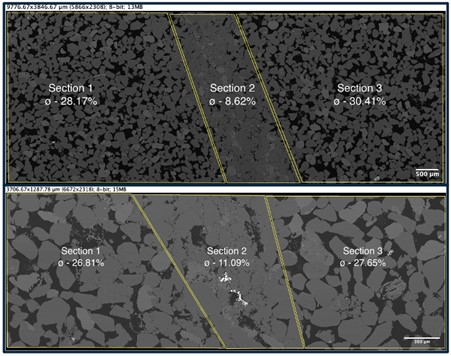
Figure 4 SEM images showing 2 sections of sandstone containing the deformation band. The porosity was calculated from the 2D image using the ImageJ software.
Imaging fluid flow at the μm-scale in mm-scale samples is not easy but fortunately one of my supervisors, Kamal, and Zaid Jangda, a post-doctoral research associate, had the requisite skills.
The main difficulty is that the stage needs to be able to rotate 360°, so all the tubing containing the gas and brine needs to be able to move with the stage, without impeding the image (see pictures below).
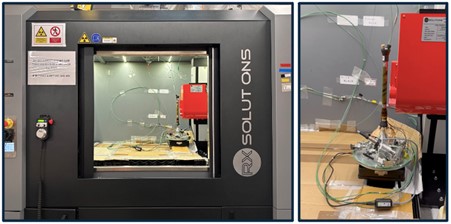
Figure 5 The CT scanner cabinet containing the X-ray source (red unit) and core holder (amber and black vertical tube) mounted upon a carousel-stage with a number of tubes for injecting gas and brine attached (slightly Heath-Robinson-esque).
After scanning a dry (air-filled) mini-core and a flooded (brine-filled) mini-core, we scanned after each injection of H2 (drainage) and brine (imbibition). This cycle was performed twice to simulate 2 cycles of injection and recovery in UHS.
We repeated the experiment using helium, instead of H2, to determine whether helium could be used as an analogue for hydrogen in this type of fluid flow experiment.
What’s next?
First, I need to process the data from the fluid flow experiment and ascertain the impact of deformation upon the flow of hydrogen and brine in this case. The results will then be synthesised with other deformation band research so that the effect can be magnified and a range of possible outcomes at a larger scale (decimetre to km) can be examined. Part of the scaling-up process will be to construct a model using an outcrop containing similar properties to the sandstone from the Saughall Massie borehole. I have identified an outcrop at Frodsham Hill, Cheshire that is suitable for this purpose, which I will scan and convert to a rock model before introducing the dynamic, fluid flow component.
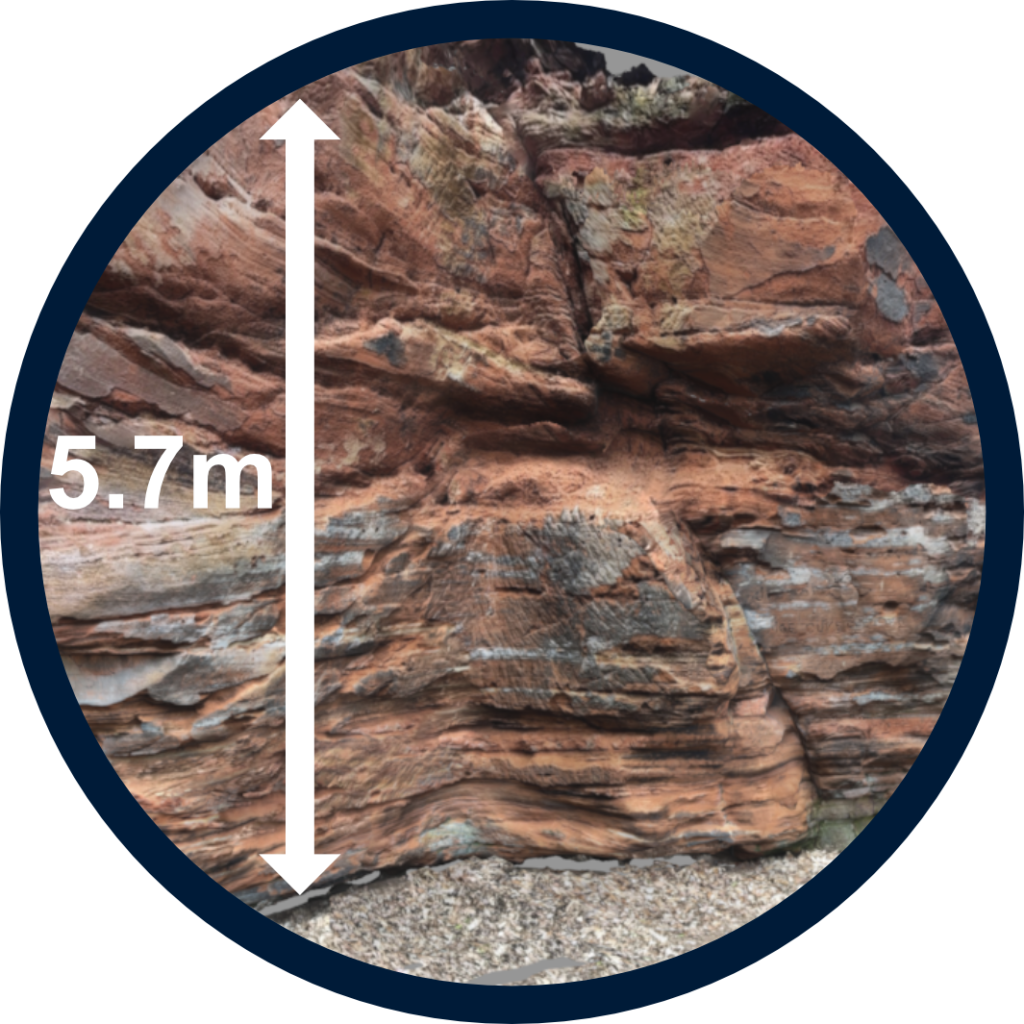
References
AusGov (2019) Australia’s National Hydrogen Strategy [online]. https://www.dcceew.gov.au/sites/default/files/documents/australias-national-hydrogen-strategy.pdf.
de Coninck, H., Revi, A., Babiker, M., Bertoldi, P., Buckeridge, M., Cartwright, A., Dong, W., Ford, J., Fuss, S., Hourcade, J. C., Ley, D., Mechler, R., Newman, P., Revokatova, A., Schultz, S., Steg, L., & Sugiyama, T. (2018). Strengthening and Implementing the Global Response. In Global warming of 1.5°C: Summary for policy makers (pp. 313-443). IPCC – The Intergovernmental Panel on Climate ChangeGriffiths (2016)
IEA (2021) Hydrogen: Tracking report – November 2021 [online]. Available at https://www.iea.org/reports/hydrogen.
IEA (2023) Global Hydrogen Review [online]. Available at https://www.iea.org/reports/global-hydrogen-review-2023.
Medici, G., Jared West, L., Mountney, N.P. (2018) Sedimentary flow heterogeneities in the Triassic U.K. Sherwood Sandstone Group: Insights for hydrocarbon exploration, Geological Journal. 2018; 1–18.
Medici, G., Jared West, L., Mountney, N.P., Welch, M. (2019) Permeability of rock discontinuities and faults in the Triassic Sherwood Sandstone Group (UK): insights for management of fluvio-aeolian aquifers worldwide, Hydrogeology Journal (2019) 27:2835–2855, https://doi.org/10.1007/s10040-019-02035-7.
Newell, A. J., Shariatipour, S. M. (2016) Linking outcrop analogue with flow simulation to reduce uncertainty in sub-surface carbon capture and storage: an example from the Sherwood Sandstone Group of the Wessex Basin, UK, Geological Society, London, Special Publications, Vol 436, pp 231 – 246, https://doi.org/10.1144/sp436.2.
Pourmalek, A., Newell, A. J., Shariatipour, S. M., Butcher, A. S., Milodowski, A. E., Bagheri, M., Wood, A. M. (2021) Deformation bands in high-porosity sandstones: Do they help or hinder CO2 migration and storage in geological formations? International Journal of Greenhouse Gas Control 107 (2021) 103292, https://doi.org/10.1016/j.ijggc.2021.103292.
RAG (2023) Underground Sun Storage 2030 [online]. Available at https://www.uss-2030.at/en/.
Smith, D., Arnold, D., Hough, E., Busch, A. (2024) Numerical modelling of the effects of permeability contrasts on underground hydrogen storage in sandstone reservoirs, Geoenergy, https://doi.org/10.1144/geoenergy2023-039.
UKGov (2021) UK Hydrogen Strategy [online]. Available at https://www.gov.uk/government/publications/uk-hydrogen-strategy.
USGov (2021) The Long-Term Strategy of the United States: Pathways to Net-Zero Greenhouse Gas Emissions by 2050 [online]. Available at https://www.whitehouse.gov/wp-content/uploads/2021/10/US-Long-Term-Strategy.pdf.
Wakefield, O.J.W., Hough, E., Hennissen, J.A.I., Thompson, J., Cripps, C., Parkes, D. (2022) Lithofacies control on the formation of deformation bands: an example from the Sherwood Sandstone Group (Induan–Anisian, Lower Triassic) in Western England, AAPG Bulletin (2022) 106 (6): 1301–1324, https://doi.org/10.1306/02032218027.